Innovative lipids for your Nucleic Acid Delivery research
The history and evolution of Nucleic Acid Delivery systems
mRNA and liposomes were both first discovered in the 1960s, and liposomes were used to deliver mRNA into eukaryotic cells in 1978.1,2 Even with the ability to inject mRNA into cells, there were several technical challenges to overcome before mRNA could be used as a therapeutic, primarily the rapid degradation of mRNA once injected into the body. In 1990, naked mRNA was injected into the muscles of rats to prove that in vivo direct gene transfer was possible.3 As early as 1995 the first mRNA vaccine carrying cancer antigens was reported.4 And more recently, lipid nanoparticles (LNPs) have been used to deliver mRNA capable of fighting SARS-CoV-2, as well as CRISPR/Cas-9 gene editing technologies.
Our understanding of mRNA and its delivery has evolved substantially in the past few decades - from naked mRNA delivery to liposomal delivery and then LNPs. We are far from where we started and probably equally as far away, if not farther, from where we are headed.
Not only do delivery systems change, but their individual components are constantly evolving to meet current challenges and demands. To date, LNPs have primarily been formulated using the following components:
WHITEPAPER: Innovative lipids for Nucleic Acid Delivery
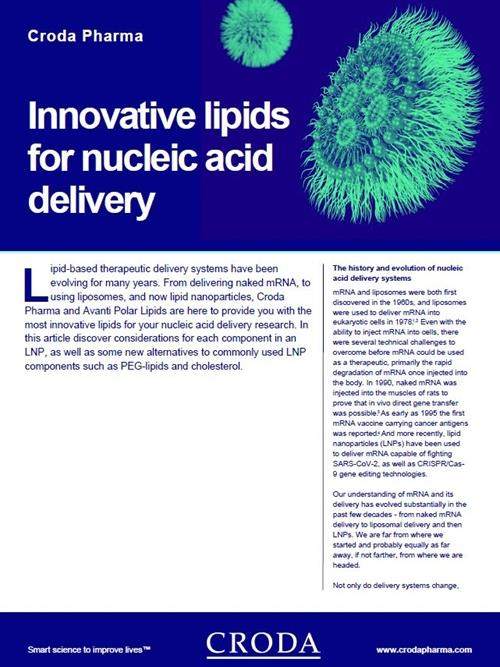
Recent research has been directed at finding new, innovative ways to improve encapsulation and transfection efficiency, and stability, as well as reduce systemic toxicity of LNP therapeutics. Keep reading to see how LNP components are evolving and where LNP technology is headed!
Considerations for future cationic lipids
In LNP formulations, cationic ionizable lipids are used to complex negatively charged mRNA. Aside from playing a major role in the LNP formulation itself, cationic lipids also serve a major role in the biological administration of mRNA to target cells. The RNA-loaded LNP fuses with the cell membrane and is then delivered into the cytosol. To be able to play these roles efficiently, a cationic ionizable lipid must be engineered with a suitable apparent acid dissociation constant (pKa). The apparent pKa of a cationic ionizable lipid is the likely pKa at the LNP surface. Currently, the cationic ionizable lipids in FDA-approved therapeutics all have an apparent pKa between 6-7. This is crucial for the cationic ionizable lipid to maintain a neutral charge while in systemic circulation (pH above the pKa of the lipid, pH ~7.5), as well as its ability to become positively charged in the endosome (pH ~6.5) and facilitate membrane fusion and subsequent cytosolic release.5
As crucial components in LNPs, new cationic ionizable lipids for nucleic acid delivery are being investigated to optimize mRNA complexation, endosomal membrane fusion and cargo release into the cytosol. Stay up to date on Avanti’s cationic lipid offerings here.
PEGylated lipids and the future of stealth agents in LNPs
Historically, polyethylene glycol (PEG)-lipids have been the polymer-lipid conjugate of choice for LNP formulations. PEG-lipids are used to prevent proteins from binding to LNPs and increase systemic circulation times, mediate indirect targeting capabilities of LNPs, promote LNP self-assembly, and control LNP size and stability. The ability of LNPs formulated with PEG-lipids to bypass the reticulo-endothelial clearance system and stay in systemic circulation gives them their nickname - “stealth agents”.5
As great as PEG-lipids are, they also present a few challenges. These challenges are commonly referred to as the “PEG dilemma”:6
- Steric hindrance of long PEG chains hinders the ability of a cell to uptake the therapeutic
- PEGylation also hinders endosomal escape of nanoparticles, leading to decreased activity of the delivery system
- Repeated administration of PEGylated systems can result in a phenomenon called accelerated blood clearance (ABC)
Other alternatives to PEG-lipids in LNPs are being explored, and recent work has identified polysarcosine (pSar)-lipids as a promising alternative. pSar-lipids, a polymer-lipid conjugate based on the amino acid sarcosine, have demonstrated similar stealth properties to PEG-lipids. Previous studies have also shown polysarcosine lipids to induce a lower immunogenic response than PEG-lipids when administered to rabbits, zebrafish embryos, and mice. And most recently, pSar-lipids were evaluated in mRNA-LNPs and displayed high RNA transfection ability and an improved safety profile.7 Avanti is now offering pSar-lipids with varying polymeric chain lengths and lipid chain lengths for your PEG-free mRNA delivery research!
Cholesterol and its structural analogs
Another major component of LNPs, making up roughly 35-45% of the final formulation, is cholesterol. Cholesterol works as a stability enhancer by filling gaps in the lipid layer and assists in the transfection of RNA. Cholesterol must be present to have an effective lipid-based delivery system but using too much cholesterol can prevent particle formation.
Since cholesterol makes up such a large portion of an LNP formulation, it makes sense that current research efforts would attempt to find more effective alternatives. With so many naturally occurring analogs of cholesterol available, evaluating the substitution of cholesterol with its structural analogs was a great starting point for a collaboration between Moderna and Oregon State University. They evaluated how three groups of steroids, 9,10-secosteroids, C-24 alkyl, and pentacyclic steroids, affected the particle size, mRNA encapsulation efficiency, and transfection efficiency of the resultant LNP formulations.7
Of the evaluated cholesterol analogs, β-sitosterol proved to be head and shoulders above the rest. β-sitosterol LNPs had a comparable particle size (diameter ~100 nm) and encapsulation efficiency (~95% using 200 ng mRNA) as the cholesterol control. Where the β-sitosterol-substituted LNPs stood out was their transfection efficiency which was several-fold higher than the cholesterol control. So, comparable size and encapsulation efficiency, coupled with markedly improved transfection efficiency makes β-sitosterol an intriguing alternative to cholesterol in future RNA-LNPs.8 Avanti now has β-sitosterol (synthetic from plant sterol) available in the research catalog. Check it out today!
LNPs are being evaluated in research labs across the globe as therapeutic delivery systems to diagnose, prevent, or fight a number of diseases. As you encounter new delivery challenges, Avanti will be here to offer innovative lipids to tackle those challenges head on. If you have a product-specific question or need to discuss a custom project with one of our experts, click here to get in touch.
2. Ostro M.J., Giacomoni D., Lavelle D., Paxton W., Dray S. Evidence for translation of rabbit globin mRNA after liposome-mediated insertion into a human cell line. Nature. 1978;274:921–923.
3. Wolff JA, Malone RW, Williams P, Chong W, Acsadi G, Jani A, Felgner PL. Direct gene transfer into mouse muscle in vivo. Science. 1990 Mar 23;247(4949 Pt 1):1465-8. doi: 10.1126/science.1690918. PMID: 1690918.
4. Conry RM, LoBuglio AF, Wright M, Sumerel L, Pike MJ, Johanning F, Benjamin R, Lu D, Curiel DT. Characterization of a messenger RNA polynucleotide vaccine vector. Cancer Res. 1995 Apr 1;55(7):1397-400. PMID: 7882341.
5. Hald Albertsen C, Kulkarni JA, Witzigmann D, Lind M, Petersson K, Simonsen JB. The role of lipid components in lipid nanoparticles for vaccines and gene therapy. Adv Drug Deliv Rev. 2022 Sep;188:114416. doi: 10.1016/j.addr.2022.114416. Epub 2022 Jul 3.
6. Fang Y, Xue J, Gao S, Lu A, Yang D, Jiang H, He Y, Shi K. Cleavable PEGylation: a strategy for overcoming the "PEG dilemma" in efficient drug delivery. Drug Deliv. 2017 Dec;24(sup1):22-32. doi: 10.1080/10717544.2017.1388451.
7. Sara S. Nogueira, Anne Schlegel, Konrad Maxeiner, Benjamin Weber, Matthias Barz, Martin A. Schroer, Clement E. Blanchet, Dmitri I. Svergun, Srinivas Ramishetti, Dan Peer, Peter Langguth, Ugur Sahin, and Heinrich Haas. ACS Applied Nano Materials 2020 3 (11), 10634-10645. DOI: 10.1021/acsanm.0c01834
8. Patel S, Ashwanikumar N, Robinson E, Xia Y, Mihai C, Griffith JP 3rd, Hou S, Esposito AA, Ketova T, Welsher K, Joyal JL, Almarsson Ö, Sahay G. Naturally-occurring cholesterol analogues in lipid nanoparticles induce polymorphic shape and enhance intracellular delivery of mRNA. Nat Commun. 2020 Feb 20;11(1):983. doi: 10.1038/s41467-020-14527-2. Erratum in: Nat Commun. 2020 Jul 6;11(1):3435. PMID: 32080183; PMCID: PMC7033178.